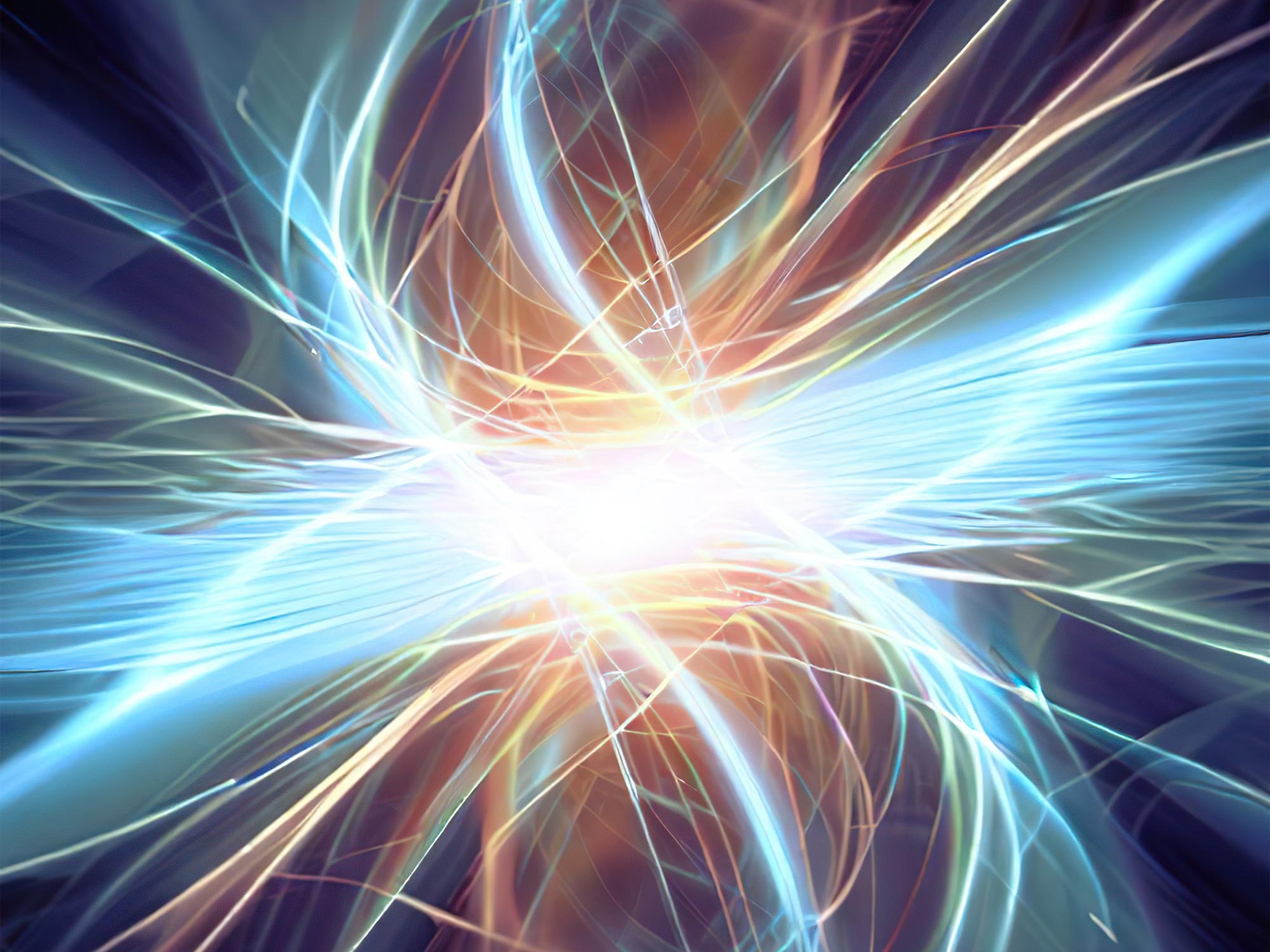
Fizycy z Penn State odkryli globalną interakcję w układach kwantowych, gdy są one zakłócane przez duży przepływ energii. Wykorzystując bardzo zimne, jednowymiarowe gazy, byli w stanie dokładnie monitorować tę reakcję i następującą po niej fazę znaną jako „hydrodynamika”, dostarczając model do zrozumienia podobnych układów kwantowych. Wyniki zostały opublikowane w czasopiśmie Natura.
Nowe eksperymenty z bardzo zimnymi gazami atomowymi rzuciły światło na ewolucję wszystkich oddziałujących układów kwantowych po nagłym napływie energii.
Nowe eksperymenty wykorzystujące jednowymiarowe gazy bardzo zimnych atomów ujawniają uniwersalność tego, jak układy kwantowe złożone z wielu cząstek zmieniają się w czasie po tym, jak duży napływ energii wytrąca układ z równowagi. Zespół fizyków z Penn State wykazał, że gazy te reagują natychmiast, „ewoluując” z cechami wspólnymi dla wszystkich „wieloczłonowych” układów kwantowych wytrąconych w ten sposób z równowagi. Artykuł opisujący eksperymenty został opublikowany 17 maja 2023 r. w czasopiśmie Natura.
„Wiele z głównych osiągnięć fizyki w ciągu ostatniego stulecia dotyczyło zachowania układów kwantowych z wieloma cząstkami” – powiedział David Weiss, wybitny profesor fizyki w Penn State i jeden z liderów zespołu badawczego. „Pomimo zawrotnej liczby różnorodnych zjawisk „wielu ciał”, takich jak nadprzewodnictwo, nadciekłość i magnetyzm, odkryto, że ich zachowanie w pobliżu równowagi jest często na tyle podobne, że można je pogrupować w niewielką grupę uniwersalnych kategorii. przeciwnie, zachowanie systemów dalekich od równowagi doprowadziło do kilku takich ujednoliconych opisów”.
Nowe eksperymenty z ekstremalnie zimnymi gazami atomowymi ujawniają uniwersalną fizykę w dynamice układów kwantowych. Absolwentka Penn State, Yuan Li, pierwsza autorka artykułu opisującego eksperymenty, stoi w pobliżu urządzenia, którego użyła do stworzenia i zbadania jednowymiarowych gazów bliskich zera absolutnego. Źródło: David Weiss, Penn State
Weiss wyjaśnił, że te wielociałowe układy kwantowe to zbiory cząstek, takich jak atomy, które mogą swobodnie poruszać się względem siebie. Kiedy są mieszaniną wystarczająco gęstych i chłodnych, które mogą się różnić w zależności od kontekstu, mechanika kwantowa – podstawowa teoria opisująca właściwości przyrody w skali atomowej lub subatomowej – jest wymagana do opisania ich dynamiki.
Układy masywnie nierównowagowe są rutynowo tworzone w akceleratorach cząstek, gdy pary ciężkich jonów zderzają się z prędkością bliską prędkości światła. Kolizje skutkują a[{” attribute=””>plasma—composed of the subatomic particles “quarks” and “gluons”—that emerges very early in the collision and can be described by a hydrodynamic theory—similar to the classical theory used to describe airflow or other moving fluids—well before the plasma reaches local thermal equilibrium. But what happens in the astonishingly short time before hydrodynamic theory can be used?
“The physical process that occurs before hydrodynamics can be used has been called ‘hydrodynamization,” said Marcos Rigol, professor of physics at Penn State and another leader of the research team. “Many theories have been developed to try to understand hydrodynamization in these collisions, but the situation is quite complicated and it is not possible to actually observe it as it happens in the particle accelerator experiments. Using cold atoms, we can observe what is happening during hydrodynamization.”
The Penn State researchers took advantage of two special features of one-dimensional gases, which are trapped and cooled to near absolute zero by lasers, in order to understand the evolution of the system after it is thrown of out of equilibrium, but before hydrodynamics can be applied. The first feature is experimental. Interactions in the experiment can be suddenly turned off at any point following the influx of energy, so the evolution of the system can be directly observed and measured. Specifically, they observed the time-evolution of one-dimensional momentum distributions after the sudden quench in energy.
“Ultra-cold atoms in traps made from lasers allow for such exquisite control and measurement that they can really shed light on many-body physics,” said Weiss. “It is amazing that the same basic physics that characterize relativistic heavy ion collisions, some of the most energetic collisions ever made in a lab, also show up in the much less energetic collisions we make in our lab.”
The second feature is theoretical. A collection of particles that interact with each other in a complicated way can be described as a collection of “quasiparticles” whose mutual interactions are much simpler. Unlike in most systems, the quasiparticle description of one-dimensional gases is mathematically exact. It allows for a very clear description of why energy is rapidly redistributed across the system after it is thrown out of equilibrium.
“Known laws of physics, including conservation laws, in these one-dimensional gases imply that a hydrodynamic description will be accurate once this initial evolution plays out,” said Rigol. “The experiment shows that this occurs before local equilibrium is reached. The experiment and theory together, therefore, provide a model example of hydrodynamization. Since hydrodynamization happens so fast, the underlying understanding in terms of quasi-particles can be applied to any many-body quantum system to which a very large amount of energy is added.”
Reference: “Observation of hydrodynamization and local prethermalization in 1D Bose gases” by Yuan Le, Yicheng Zhang, Sarang Gopalakrishnan, Marcos Rigol and David S. Weiss, 17 May 2023, Nature.
DOI: 10.1038/s41586-023-05979-9
In addition to Weiss and Rigol, the research team at Penn State includes Yuan Le, Yicheng Zhang, and Sarang Gopalakrishnan. The research was funded by the U.S. National Science Foundation. Computations were carried out at the Penn State Institute for Computational and Data Sciences.
„Całkowity miłośnik kawy. Miłośnik podróży. Muzyczny ninja. Bekonowy kujon. Beeraholik.”
More Stories
Prognoza cukrzycy w Australii w 2024 r. | Wiadomości o Mirażu
„Gorąca sauna żabia” pomaga australijskim gatunkom w walce ze śmiercionośnym grzybem
Model sztucznej inteligencji poprawia reakcję pacjentów na leczenie raka